Laser Micromachining Systems 금속재료 미세가공 시스템
-
Laser micromachining can be used to drill, mill, and cut metals with micron precision.
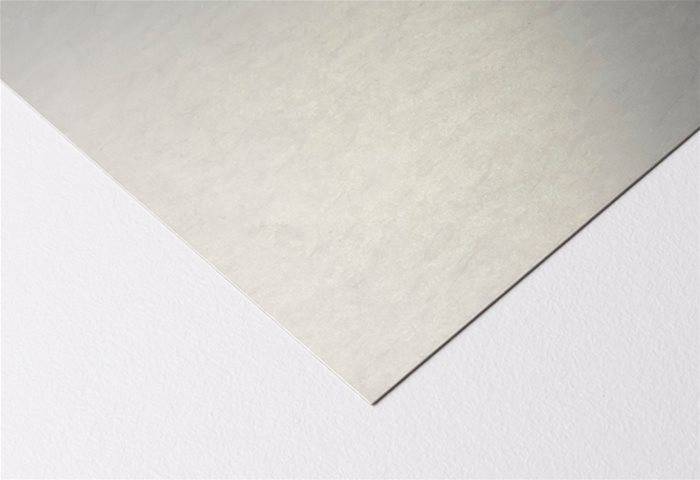
All metals can be laser processed with features sizes in the range of single microns through to hundreds of microns.
Laser micromachining can be used to machine a wide range of metals which include stainless steel, copper, aluminum, and gold etc. As well as these more common metals, Oxford Lasers specialize in machining materials that are particularly difficult to machine using conventional means such as high melting point metals like tungsten, tantalum, iridium and molybdenum, these metals being particularly difficult to machine conventionally due to their high hardness level.
Oxford Lasers have a wide variety of lasers sources to process different metals, which allow us to overcome limitations of burr formation, melting, crack formation etc.
All metals can be machined where parts have overall lengths in centimeters, with laser micromachining particularly valuable in processing feature sizes of <250microns; for features sizes <100microns, laser micromachining truly shines. MORE DETAILS
A sample of metals we can machine include:
Aluminium | Copper | Gold | Steel | Tungsten | Plus many more...
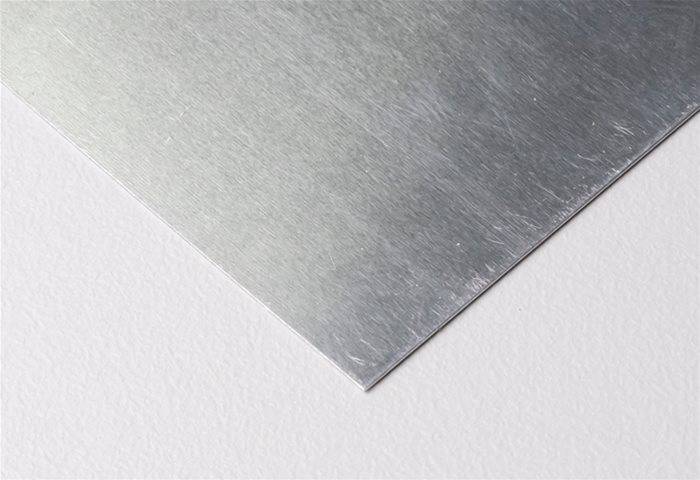
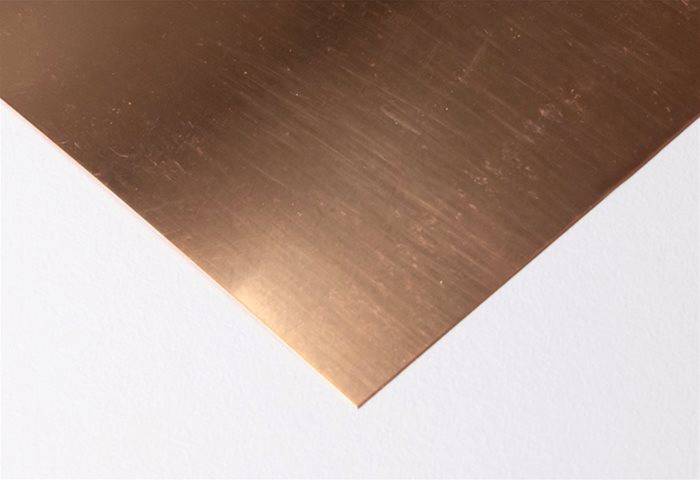
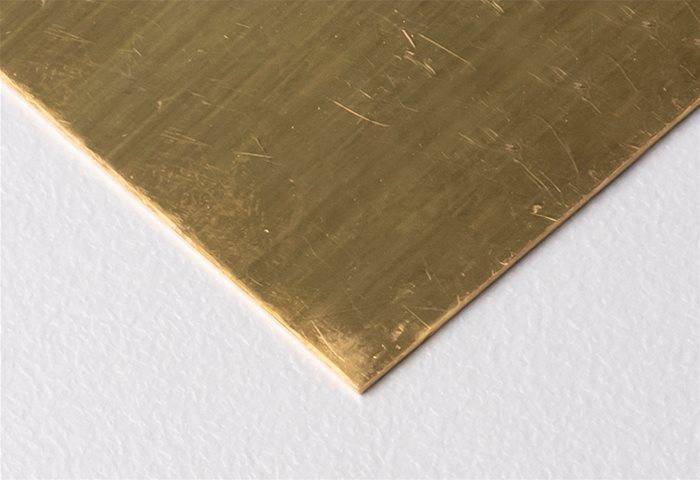
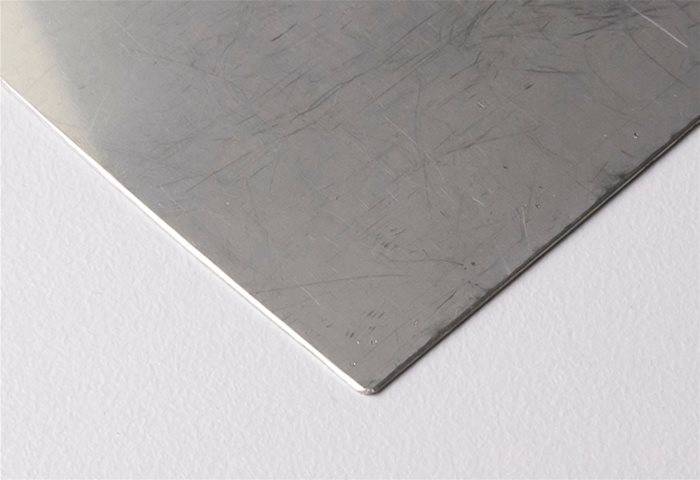
Spray Characterization - Laser Imaging System : VisiSize N60 system
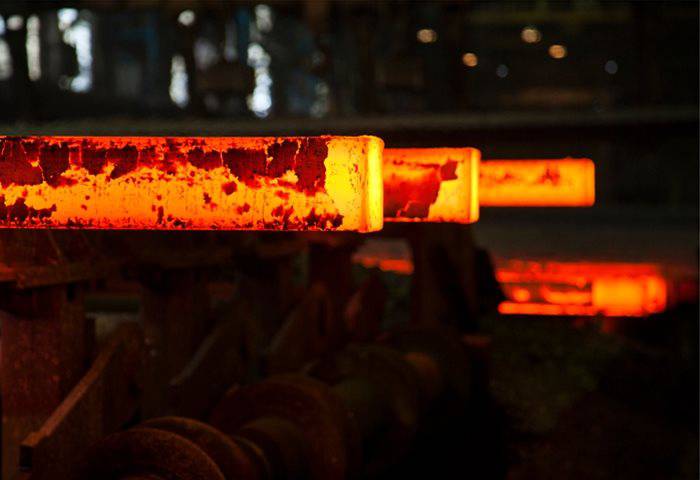
- Hot-rolled steel coil manufacture
- Industrial Nozzle Characterization
It is the microstructural constituants of the material that define the mechanical properties of steel, namely the strength, hardness and dislocation density of the material. The cooling rate of steel during the production process after the final deformation stage has a large impact on the mechanical properties of the material as well as the shape and final flatness of the product.
Air atomised sprays are widely used within the industry to control this cooling rate as the high velocity of the spray droplets generated through the use of compressed air with the liquid flow enables the liquid to get close to the surface of the material to remove the high-density heat fluxes from the solid surfaces.
The size of the parts generated through the rolled steel production of billets of steel for example require the use of multiple nozzles. Therefore understanding of the nature of the drop size distribution and volume flux across the application area is critical to the development of a homogenous cooling process and therefore developing a uniform grainsize distribution throughout the product. The drop size measurement capability of the VisiSize N60 system enables the determination of the drop size distribution variation across the spray application area. This can be done in-situ of the instrumentation as the system has a Class 1 laser safe classification.
Oxford Lasers provide products and services to a wide variety of industries for in-line production imaging analysis.
철 및 비철 금속 성분 분석기 (XRF)
금속 재료 성분 분석기 (Arc Spark-OES)
Ferrous Metallurgy
X-ray fluorescence energy dispersive general purpose spectrometer BRA-135F
Ferrous metallurgy includes production of cast iron (blast-furnace process) and, using it as a base, production of plain, low- and high-allow steels and ferro-alloys. In blast-furnace process, the main materials to be analyzed are iron concentrate, coke, and fluxes (incoming inspection). Mg, Al, Si, Ca are to be detected both in concentrate and fluxes in addition to Fe.
The detrimental impurity – sulphur – is of primary importance in coke. As a rule, powdered samples are subject to analysis. At the blast-furnace process output, cast iron is subject to Mn, Cr, Ni, Ti, Si, S, P and carbon, which is detected through combustion. To control the process, slags are analyzed for Mg, Al, Si, Ca, Fe.
Manganese and chrome ores are processed to obtain ferro-alloys (ferromanganese and ferrochrome) used for alloying steels where the content of basic components and additives Si, Ti and S is controlled. Other chemical elements used for steels alloying are also used as corresponding ferro-alloys, which composition is strictly controlled to maintain the composition of the furnace charge of the steel grade being made.
Since certain elements are burnt and turn into slag when alloyed steels are made, the analysis of the melting progress is to be performed to bring the melt composition in compliance with that of the required grade. The only method ensuring the proper rapidity and accuracy of steels analysis in the course of melting is XSFA. Depending on the steel grade being made, alloying additives (which may include Ti, V, Cr, Mn, Co, Ni, Nb, Mo and W) usually introduced as ferro-alloys or secondary raw materials are determined. The content of these elements may vary from n×10-1 to 20 – 30%, depending on the steel grade. The melt sample is taken into a water-cooled copper mold. After the sample gets solid, it is cut, polished and sent to the laboratory via a pneumatic tube. The whole process, including the analysis, takes less than 10 minutes. If there are standard samples of all grades being made at the production facility, any method may be used for calculation of concentrations (if there are no proper standard samples, methods the theoretical correction or fundamental parameters may be used). After melting is completed, marking analysis is performed to check the quality of finished products, and results thereof along with the finished product are handed over to the consumer. Methods of steel XSFA are described in various regulatory documents. Some of them are given below:
1. GOST 28033-89. Steel. X-ray fluorescence analysis method.
2. ASTME1085-09. Standard method of low-alloy steel analysis by means of X-ray fluorescence spectroscopy.
3. ASTM E572. - 02a(2006)e2. Standard method of stainless and low-alloy steel analysis by means of X-ray fluorescence spectroscopy.
4. ISO 17054:2010. Routine method of high-alloy steel analysis by means of X-ray fluorescence spectroscopy and using the method of corrections.
General-Purpose X-ray diffractometers DRON-7(M) and DRON-8
X-ray diffractometers DRON-7 and DRON-8 are successfully used for qualitative and quantitative analysis of phase composition of ferrous and non-ferrous metals and alloys, as well as for detection of retained austenite in high-carbon steels; for control of metallurgical production waste (slags); for examination of grain patterns of rolled metal and for analysis of residual stress in steelworks.
Non-ferrous Metallurgy
X-ray fluorescence energy dispersive general purpose spectrometer BRA-135F
As far as non-ferrous metallurgy is concerned, the metallurgical process may result in both pure metals and corresponding ferro-alloys or oxides and some metal compounds. As a rule, sulphide nonferrous metal ores (Co, Ni, Cu, Zn, Pb, Mo) are used most frequently, and for metal smelting sulphide concentrates previously separated by floatation are used. The first stage of sulphide concentrates processing is oxidizing flux roasting in reverberatory furnaces, with metal sulphides turning over into corresponding oxides. Roasting results are controlled by analysis. Further reducing roasting allows obtaining crude metals - Co, Ni, Cu, Zn, and Pb to be refined through electrolytic process. This method allows separating precious metals which are often present in detectable amounts in complex ores.
As far as metals used for steel alloying are concerned, reducing roasting with cast iron or iron concentrate and coke is used, which results in production of corresponding ferro-alloys (Ni, Mo, W). Rare metals Mo and W are processed to obtain oxides MoO3 and WO3, which are refined and used to obtain pure metals or their compounds.
Like during production of ferrous metals, fluxes and slags are analyzed during smelting of non-ferrous metals and detected for Mg, Al, Si and Ca in addition to smelted metals.
Complex processes of ferrous and non-ferrous metals production using concentrates of complex ores imply multiple analysis of early, intermediate and final products not only for base metals but also for numerous additives covering almost the whole Mendeleev's table, starting from Al, Si, S and P and ending with platinum-group metals, Au and Bi.
Use of BRA-135 is also possible and appropriate at separate stages of production of light metals (Mg, Al, Ti), for example, for control of the content of basic components and additives in ores and concentrates of these metals.
Among non-ferrous alloys, alloys based on copper (brass and bronze) and aluminum alloys are used most frequently. In addition to copper, copper-based alloys may include Zn, Ni, Fe, Mn, Sn, Pb, Bi, Si, P, Sb, Al, Be. Among all these metals only Be in beryllium bronzes cannot be detected by the XSFA method.
XSFA methods of alloys based on copper (bronzes and brasses) are described in various regulatory documents. Some of them are given below:
1. GOST 30609-98 Cast brasses. X-ray fluorescence analysis method.
2. GOST 20068.4-88. Tinless bronzes. Method of X-ray spectrum detection of aluminium.
3. GOST 30608-98. Tin bronzes. X-ray fluorescence analysis method.
Aluminium alloys may include Mg, Ti, Cr, Mn, Fe, Cu, Zn, Zr, Sn, Pb.
However one should emphasize that the method of fundamental parameters may have significant errors when applied to non-ferrous alloys often containing lead, due to micro-absorption discontinuity (lead is poorly soluble in copper and aluminium, thus producing individual inclusions). Because soft lead is spread over the surface during preparation of non-ferrous alloy samples, lathe machining rather than grinding is used.
XSFA is one of the main analysis methods for rare metal alloys that may include Al, Ti, V, Co, Ni, Zr, Nb, Mo, Nb W, Re and some additives. XSFA methods for rare metal alloys are described in regulatory documents:
1. GOST 28817-90. Hard sintered alloys. X-ray fluorescence method of metals detection.
2. GOST 25278.15-87. Alloys and addition alloys of rare metals. X-ray fluorescence method of detection of zirconium, molybdenum, tungsten and tantalum in niobium-based alloys.
3. ASTM B890 - 07(2012). Standard Test Method for Determination of Metallic Constituents of Tungsten Alloys and Tungsten Hardmetals by X-Ray Fluorescence Spectrometry.
4. ASTME539-07. Test Method for X-Ray Emission Spectrometric Analysis of 6Al-4V Titanium Alloy.
5. ASTM E2465-06. Standard Test Method for Analysis of Ni-Base Alloys by X-ray Fluorescence Spectrometry.
As with steels, XSFA is performed in the course of smelting, marking analysis of the finished product and incoming inspection using various analytical methods are performed.
General-Purpose X-ray diffractometers DRON-7(M) and DRON-8
X-ray diffractometers DRON-7 and DRON-8 are successfully used for qualitative and quantitative analysis of phase composition of ferrous and non-ferrous metals and alloys, as well as for detection of retained austenite in high-carbon steels; for control of metallurgical production waste (slags); for examination of grain patterns of rolled metal and for analysis of residual stress in steelworks.
Jewellery Alloys
X-ray fluorescence energy dispersive general purpose spectrometer BRA-135F
Use of XSFA for analysis of jewellery alloys and items made thereof by means of the energy dispersion X-ray spectrometer Shimadzu, which is similar to BRA-135, is described in Application Note at the company’s site. Co, Ru, Rh, Pd, Ag, Pt, Au were detected. The content of precious metals in analyzed items equalled to 7 to 85%. A zirconium filter was used for measurements, with the 1 mm original radiation collimation. The analysis error equalled to 0.7 to 0.25%. The work by А. V. Fesenko and Н. Г. Milozorov [RUSSIAN JOURNAL OF GENERAL CHEMISTRY, 2002, vol. XLVI, №4, p.81-87.] has compared possibilities of existing analysis methods for gold alloys, including XSFA. General requirements to analysis of precious metals and their allows are described in the standard [GOST R 52599-2006. Precious metals and their alloys. General requirements to analysis methods].
Machine-building
X-ray fluorescence energy dispersive general purpose spectrometer BRA-135F
The fist and one of the most important stages of XSFA use is incoming inspection of all the metals and alloys coming the machine-building plant. Unfortunately, this stage is often neglected, and the supplier’s supporting documentation is relied on. Rare mistakes are found only in case of failure of finished item, which leads to significant losses. A typical example thereof is supply of carbon steel pipes to Leningrad mechanical plant in 1979, without incoming inspection of escalator rollers used for manufacture, instead of chromium steel pipes. The mistake was revealed only when several cracking rollers were analyzed.
Similar cases occurred even in aerospace industries of the USA and USSR.
Incoming inspection of alloys may be performed by means of XSFA similarly to the supplier’s marking analysis, or as long as samples of all the alloys used at the enterprises are available, by means of program IDENTIFICATION-W developed in Bourevestnik, Inc. The case when a part of dubious composition has been already included into the finished item is more complicated.
Sometimes portable manual X-ray fluorescence spectrometers based on SSD may be used for composition control of such item, but the most universal method is treating the part with an abrasive paper. Alloy traces remaining on the paper may be compared to those on the paper used for treating a sample steel of the proper grade, using spectrometer BRA-135 and program IDENTIFICATION-W.
Another important task solved by energy dispersion X-ray spectrometers in machine-building is control of coating thickness and composition. Some regulatory documents on the topic are given below:
1. ASTM B568-98(2009). Standard Test Method for Measurement of Coating Thickness by X-Ray Spectrometry.
2. ISO 3497:2000 Metallic coatings -- Measurement of coating thickness -- X-ray spectrometric methods.
3. JIS H 8501-1999 Methods of thickness test for metallic coatings.
Depending on the coating and substrate composition, the coating thickness may be controlled within the range from tenths and hundredths of micron to dozens of microns. An experimental model of the X-ray energy dispersion thickness gauge of gold coatings was manufactured by Bourevestnik as early as in 1974 and tested on gold coatings of one of watchmakers. It turned out that in some cases the coating thickness reached 4 µm instead of 10 µm as per the specification.
General-Purpose X-ray diffractometers DRON-7(M) and DRON-8
The method of seeded directional crystallization has found a wide use in production of turbine buckets for aircraft engines all over the world since it allows producing defect-free single-crystal buckets made of heat-resistant alloys. To analyze the direction of seeds and grown buckets, X-ray diffraction method employing diffractometers DRON-7 and DRON-8 operating in sorting mode has been used successfully.
This method may be used for control of direction of single-crystal casts in machine-building production.
The X-ray diffraction method for analysis of residual stresses in various parts of machines and mechanisms is also wide-spread in machine-building.
금속성분분석기 (AAS)
3D Profilometer (3차원 형상분석기)
Universal Testing Machine (UTM) from Testresources, USA
Metals are hard, strong and tough and by their nature, are malleable, fusible and ductile which means that they can be formed into a vast array of desired shapes without cracking or breaking. Due to their nature they can be treated to alter characteristics and formed to meet the requirements of any number of applications.
Metal Testing Goal:
Testing the mechanical properties of metallic materials determines if it meets the requirements of a desired application. The most common characteristics measured during testing are the modulus of elasticity, yield strength, tensile and compressive strength, and elastic limit. Metals are also tested to measure modulus of rigidity, shear strength, bend strength, flexure strength and fatigue strength as well as the time dependent behavior such as creep and stress relaxation. These values provide an accurate depiction of the behavior that a metal material may be expected to exhibit during loading and the lifetime of its application.
Common Metal Test Methods:
Test methods for metals tend to be very similar as those used for the testing of other materials such as plastics or composites. Flexure, bend, shear and torsion tests are all performed on metals, but the most common tests are the tension, compression and fatigue tests.Tension and compression testing involve the metal sample experiencing a load along one of its axis that either pulls the material apart or pushes it together. These tests are generally run until the sample fails or ruptures but may be stopped at any time.
Shear and torsion tests require that the sample experiences opposing loads at each end that act perpendicularly to the axis of the sample. A shear tests will pull the ends of the sample so it will fail in a sliding motion whereas a torsion test will twist the ends of the sample so it will fail in a turning motion.
A metal sample for flexure and bend tests will experience a load at its midsection while it is supported at its two ends. As the load is increased the sample will be deformed into a “U” or “V” shape depending upon its characteristics.
Fatigue testing measures time and cycle dependent behavior of metals through cyclic loading. These tests measure the number of cycles till failure, the time till failure, and possibly creep and stress relaxation rates of the metal samples.
All of these tests are used to determine if a metal material is suitable for an application and are often combined with each other to create an accurate representation of the stresses a metal material will experience in its lifetime.
Metal Testing Specimens:
Testing specimens made from metal can essentially take any form. They commonly come in the shape of dog bones, dumbbells, rods, bars, cylinders, cubes, bricks, and tubes. The difference between test samples made from metal and test samples made from other materials is in the microstructure. Metals can undergo processes such as heating, quenching, annealing and others that alter its microscopic composition. Specifically the grain size, grain orientation and the dislocations are altered to change the characteristics of the metal such as its strength, toughness, and ductility.
Related Articles
- Tensile Strength Test of Fibers, Threads, Wires or Nanotubes
- Torsion Testing of Wire | Equipment
- Tensile Testing Aluminum and Magnesium Metal Alloys
- Tensile Test Equipment for Rebar
- Tensile Test Machines for Steel Products
- Tensile Grips - Capstan, Pneumatic, and Wedge for Wire
- How to Plan Tensile Tests for Wire Crimp, Solder, and Lugs
- How to Configure Test Equipment for Tensile Test of Metals
- Biaxial Tension Tests of Sheet Metal
- Biaxial fracture toughness and crack growth tests of metal
- Low Cycle Fatigue (LCF) of Metals at High Temperature
- Strain Controlled Low Cycle Fatigue Testing Equipment
- Guided Bend Test of Metal Welds
- Four Steps to Select Compression Test Equipment for Metals
- Fine Wire Test Grips and Equipment
- Tensile Testing of Nitinol Wire
- Wire Tensile Testing Standards: Crimp, Solder, and Lug Wire Connections
- Wire Torsion Strength Test | Equipment
- How to pull test cable and wire products
- Cable Proof Load Limit Mechanical Test Equipment
- Metal Forging Fatigue, Fracture and Tensile Test Equipment
- Steel Reinforcing Bar or Rebar Tensile Test Equipment
- Stainless Steel Fatigue Cryogenic Tests
- Weld Strength Tests using Guided Bend and Tensile Fixtures | Equipment
- Metals Fracture Toughness Testing | Machines & Equipment
- Testing Metals for Thermo Mechanical Fatigue (TMF) Life | Equipment
- Metals Fatigue Crack Propagation Tests with Variable Amplitude Loading
- Metal Chain Static and Fatigue Test Application
- Weld Ductility Bend Test
- Metal Ductility Bend Test
- High Cycle Fatigue (HCF) of Metals
- Metals Crack Growth Planar Biaxial Mode
- Metals Testing - Axial Torsion | Equipment
- Metals Fatigue Testing at High Temperatures
- Single-Lap-Joint Adhesively Bonded Metal Specimens Apparent Shear Strength by Tension Loading
- Steel Pipeline Tensile Testing
- Rebar Tensile and Bend Testing
- Pre-stressed Concrete Multi-Wire Strand Tensile Tests
- Structural Steel Tension Testing
- Gray Iron Casting Dumbbell Samples - Tensile Testing
- Iron Pipe Tensile Testing
- Elevated Temperature Tension Tests of Metals
- Sheet Metal R and N values Testing
- Metallic Materials Tensile Testing
- Tensile Testing of Thin Metal Foil
- Tensile Testing of Metal Dogbone Specimens
- Testing Materials in Radioactive Hot Cells
- Wires, Cables and Rope Pull Testing
Applicable Standards
- ASTM A1022 Stainless Steel Wire Concrete Reinforcement Test Equipment
- ASTM A1044 Steel Stud Assemblies Test Machine
- ASTM A370 Mechanical Testing of Steel Products
- ASTM A496 Tension Bend Steel Wire Test Equipment
- ASTM A938 Torsional Test of Wire
- ASTM D1002 Lap Shear Testing for Adhesively Bonded Metals
- ASTM E1820 Fracture Toughness Test Equipment for Metals
- ASTM E190 Guided Bend Test Equipment for Ductility of Welds
- ASTM E21 Elevated Temperature Tension Tests of Metals
- ASTM E2368 Thermomechanical Fatigue Test Equipment
- ASTM E2760 Creep Fatigue Crack Growth Test Equipment
- ASTM E290 Bend Testing of Material for Ductility
- ASTM E399 Linear-Elastic Plane-Strain Fracture Toughness (K1c) Testing of Metallic Materials
- ASTM E466 Load Controlled Constant Amplitude Fatigue Tests of Metals
- ASTM E606 Strain-Controlled High and Low Cycle Fatigue Testing
- ASTM E647 Fatigue Crack Growth Test Equipment
- ASTM E8 Tension Testing of Metallic Materials
- ASTM E9 Compression Testing of Metallic Materials at Room Temperature
- ASTM F2516 Tension Testing Equipment for Nickel-Titanium Superelastic Materials
- ASTM F382 Flexural Fatigue Bone Plate Fixation Devices Test Equipment
- ASTM F384 Bending Fatigue Orthopedic Fixation Devices Test Equipment
- ISO 15630 Steel Reinforcement of Concrete
- ISO 6892-1 & 2 Metal Tensile Testing
- ISO 7800 Metal Wire Torsion test
- ANSI/AWS B4.0 Mechanical Testing of Welds
하기